Image 1. (above) shows high levels of methane over the poles. Peak levels of over 2000 ppb over Antarctica have been recorded repeatedly over the past few months, which is extraordinary, the more so since the photochemical sink for methane is typically strongest during summer, while average levels of methane over Antarctica have historically been the lowest on Earth (image 2. below).
The above image 1. also shows high levels of methane over the Arctic; very high methane levels are now commonly recorded in the Arctic. The animation below, from the Arctic-news blog, shows methane levels at various altitudes on January 23, 2013, ranging up to 2241 ppb at 742 mb.
![]() |
Image 3. Methane at up to 2241 ppb on January 23, 2013 - this is a 2.42 MB animation that may take some time to fully load |
![]() |
Image 4. IASI methane levels March 1-10, 2013, by Leonid Yurganov, against NSIDC sea ice concentration map March 12, 2013. Note: this is a 3.09 file that may take some time to fully load. |
In conclusion, at both poles methane levels can be very high, i.e. higher than elsewhere on Earth, and this methane likely originated from hydrates that have become destabilized by temperature rises.
2. How much methane is there?
![]() |
Image 5. by Jemma L. Wadham |
Image 5. on the right shows the ice margin of an Antarctic glacier, depicting frozen lake sediments in the foreground. When ice sheets form, they overrun organic matter such as that found in lakes, tundra and ocean sediments, which is then cycled to methane under the anoxic conditions beneath the ice sheet. The methane could be released to the atmosphere if the ice sheet shrinks and exposes these old sedimentary basins.
![]() |
Image 6. Conversion table |
![]() |
Image 7. The East Siberian Arctic Shelf (ESAS) |
- organic carbon in permafrost of about 500 Gt
- about 1000 Gt in hydrate deposits
- about 700 Gt in free gas beneath the gas hydrate stability zone.
By comparison, the total amount of methane currently in the atmosphere is about 5 Gt.
3. Methane's potency as a greenhouse gas
Releases of methane into the atmosphere are very worrying, given methane's high potency as a greenhouse gas.
![]() |
Image 8. IPCC and Methane's GWP (global warming potential) |
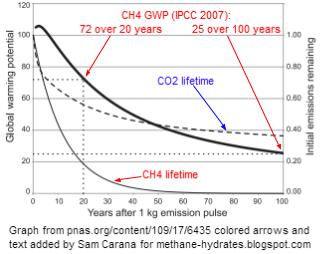
Methane's global warming potential (GWP) will rise in case of large releases, since methane's lifetime will be extended as the burden rises, due to hydroxyl depletion. This is a feedback effect that the IPCC estimates will amplify the climate forcing of an addition of methane to the current atmosphere by lengthening the perturbation lifetime relative to the global atmospheric lifetime of methane by a factor of 1.4.
A 2009 NASA article discussing the work by Drew Shindell mentions that, over the years, increases in global methane emissions have caused a 26% decrease in hydroxyl.
![]() |
Image 9. Methane's Global Warming Potential |
In its Fourth Assessment Report (AR4, 2007), the IPCC gave methane a GWP of 25 as much as carbon dioxide over 100 years, and 72 as much as carbon dioxide over 20 years.
A 2009 study by Drew Shindell et al. points out that the above IPCC figures do not include direct+indirect radiative effects of aerosol responses to methane releases that increase methane's GWP to 105 over 20 years when included.
In the context of tipping points, which is most appropriate regarding methane releases in the Arctic, it makes sense to focus on a short time horizon, i.e. as short as a few years, rather than decades.
As image 9. (left) shows, based on the figures by Shindell et al. and using a horizon of 10 years, methane's GWP is more than 130 times that of carbon dioxide.
In image 9., the blue line is based on IPCC AR4 figures. The red line is based on figures from the study by Shindell et al., which also concludes that methane's GWP would likely be further increased when including ecosystem responses.
The danger is that a large abrupt methane release in the Arctic will trigger further local releases. This danger is particularly high the first few years after the methane enters the atmosphere, due to methane's high initial warming potential, as further discussed in the next two chapters.
Meanwhile, the IPCC has further upgraded methane's lifetime and GWP, as illustrated by the image below.
![]() |
Image added October 2013, based on screenshot of IPCC document with text added by Sam Carana |
4. Methane's Local Warming Potential
Methane releases from hydrates at the poles differ in a number of ways from the methane that is typically released elsewhere around the globe:
![]() |
Image 10. Methane's Local Warming Potential |
- Abrupt release of methane from hydrates will initially be highly concentrated in one location.
Other types of methane releases (e.g. from wetlands, livestock, bio-waste and burning of fuel) occur spread out over the world throughout the year, i.e. such releases are pretty much global and continuous to start with and can be more easily broken down by hydroxyl that is continuously produced around the world.
By contrast, much of the methane from an abrupt release in the Arctic Ocean will initially remain concentrated over the Arctic Ocean, which covers only 2.8% of the Earth's surface. While the methane will eventually spread around the world, this will take time. The Jet Stream acts as a barrier, preventing air from flowing out of the Arctic and preventing air from elsewhere to enter the Arctic. Nesbit mentions that a major methane release in the high Arctic would take 15-40 years to spread to the South Pole.
Given the lack of hydroxyl in the Arctic atmosphere (see below), it may well be possible that some 20% of the methane from an abrupt release over the Arctic Ocean still remains there after 5 years. Using a GWP for methane of 130 times that of carbon dioxide over a period of ten years would already lift methane's LWP (local warming potential) over the Arctic to (130*100:2.8:5=) 929 over 10 ten years. For a shorter period, the Arctic LWP will be even higher. In conclusion, local concentration alone makes that a methane cloud still hanging over the Arctic five years after its release will have a huge LWP, i.e. well over 1000 times the potency locally that the same mass of carbon dioxide has globally.
- Additionally, high temperature anomalies make that methane releases at the poles will be felt more severely than elsewhere on Earth. These high temperature anomalies are the result of feedbacks such as albedo changes caused by melting. Image 11. below, from Skeptical Science, pictures the loss of ice mass on Antarctica.
Arctic-news blog, shows the three areas where albedo change will be felt most in the Arctic, i.e. sea ice loss, decline of albedo in Greenland and more early and extensive retreat of snow and ice cover in other areas in the Arctic.Image 12. Big changes in Arctic within years
Image 13. Image 14.
- High levels of insolation in summer. The amount of solar radiation received by the Arctic at the June Solstice is higher than anywhere else on Earth, as illustrated by image 15. below. Similarly, solar radiation in Antarctica at the December solstice is the highest on Earth.
Image 15. Insolation on the Northern Hemisphere - As mentioned above, low levels of hydroxyl will lift methane's LWP at the poles. Methane is typically broken down by hydroxyl in the atmosphere. Very little hydroxyl is present in the atmosphere over the poles, as illustrated by image 16. This makes that, in case of large abrupt methane release at the poles, the little hydroxyl that is there will soon be depleted, and the methane will hang around for much longer locally than elsewhere on Earth.
Image 16. Global hydroxyl levels at day and night. Credit: Taraborelli et al. (2011)
5. Situation particularly dangerous in the Arctic
As described in the previous chapter, the local warming potential (LWP) of methane is very high in the Arctic. This LWP is further augmented by a number of circumstances that are particular for the situation in the Arctic today:
- Soot. The Arctic is experiencing a huge increase in the amount of sunlight that is absorbed in summer, as a result of soot deposited on snow and ice. Soot is particularly prevalent on the Northern Hemisphere.
- Rivers. A number of large rivers end up in the Arctic Ocean; they can carry warm water in summer, substantially contributing to warming up of coastal waters in the Arctic.
- Albedo. The rapid decline of Arctic sea ice and show cover in the Arctic result in ever more sunlight being absorbed in the Arctic, rather than being reflected back into space, as before.
- Greenhouse gases. Levels of carbon dioxide and methane are higher at high latitudes than elsewhere on Earth.
- Wildfires. Rising temperatures make the forests, tundras and peatlands in the Arctic more prone to wildfires that, apart from adding further soot, also add further pollutants in the atmosphere such as methane and carbon monoxide that take hydroxyl out of the air, thus extending methane's lifetime.
- Shallow waters. As described in the previous chapters, the danger is that large abrupt release of methane will trigger subsequent releases, and this danger is particularly high in shallow seas that are so prominent in the Arctic (light blue areas on image 17 on the right).
Being shallow makes waters prone to warm up quickly during summer peaks, allowing heat to penetrate the seabed.
Methane rising through shallow waters will also enter the atmosphere more quickly. Elsewhere in the world, releases from hydrates underneath the seafloor will largely be oxidized by methanotroph bacteria in the water. In shallow waters, however, methane released from the seabed will quickly pass through the water column. Large abrupt releases will also quickly deplete the oxygen in the water, making it harder for bacteria to break down the methane.
Additionally, the relatively low water temperatures and long sea currents in the Arctic Ocean are not very friendly toward methanotroph bacteria that would otherwise break down methane in the water, compared to elsewhere on Earth. In the Arctic, much of the methane that is abruptly released from the seabed is therefore prone to enter the atmosphere without being broken down by microbes in the water.
Image 18. Shallow waters of the East Siberian Arctic
Shelf (ESAS)
Shakova and Semiletov warn, in a 2010 presentation, that some 75% of the East Siberian Arctic Shelf (ESAS, rectangle on image left) is shallower than 50 m.
As the Arctic ice-cap shrinks, there will be more open water, which not only absorbs more heat, but which also results in more potential for storms to cause damage to the seafloor in coastal areas such as the ESAS, where the water is on average only 45m deep.
By how much will the sea warm up during such extreme local warming events?
Image 19. Warming of coastal waters
In shallow waters, heat can more easily reach the bottom of the sea. In 2007, strong polynya activity caused more summertime open water in the Laptev Sea, in turn causing more vertical mixing of the water column during storms in late 2007, according to one study, and bottom water temperatures on the mid-shelf increased by more than 3°C (5.4°F) compared to the long-term mean.
Another study finds that drastic sea ice shrinkage causes increase in storm activities and deepening of the wind-wave-mixing layer down to depth ~50 m (164 ft) that enhance methane release from the water column to the atmosphere. Indeed, the danger is that heat will warm up sediments under the sea, containing methane in hydrates and as free gas, causing large amounts of this methane to escape rather abruptly into the atmosphere.
Image 20. Pingos and conduits. Hovland et al. (2006)
Heat can penetrate cracks and conduits in the seafloor, destabilizing methane held in hydrates and in the form of free gas in the sediments.
As said, when methane does escape from the seafloor, it can easily rise through shallow waters without getting oxidized by methanotroph bacteria. As the methane causes further warming in the atmosphere, this will contribute to the danger of even further methane escaping, further accelerating local warming, in a vicious cycle that can lead to catastrophic conditions well beyond the Arctic.
![]() |
Image 17. Shallow waters in the Arctic Ocean |
6. Changes in the Arctic
In summary, the danger is that large abrupt release of methane will contribute to high sea surface temperature in summer, mixed down to the seafloor during storms, to penetrate cracks and conduits in the permafrost, destabilizing the methane held in sediments and triggering subsequent methane releases, and this danger is particularly high in the shallow seas of the Arctic.
![]() |
Image 21. For more details on feedbacks, see extended version of this image and discussion at
|
Above image 21. illustrates how this dangerous situation has developed in the Arctic, and what can be expected in future without action. The image pictures three kinds of warming (red lines) and their main causes:
- Emissions by people cause global warming, with temperatures rising around the globe, including the Arctic. High levels of greenhouse gases in the Arctic, combined with the impact of aerosols such as soot, and rivers that end in shallow waters in the Arctic combine to cause high summer temperature peaks in the Arctic. In other words, global warming is amplified in the Arctic.
- Accelerating Arctic warming is caused by at least ten feedback effects. Melting of snow and ice causes albedo changes, i.e. less sunlight gets reflected back into space and instead gets absorbed, causing further warming. Additionally, soot, dust and volatile organic compounds settle down on snow and ice, causing further albedo change.
Three of these feedbacks are pictured as gold lines:
- Fires feedback: Accelerated warming in the Arctic is changing the Jet Stream, contributing to increased frequency and intensity of droughts and heatwaves.
- Albedo feedback: Accelerated warming in the Arctic also speeds up the decline of ice and snow cover, further accelerating albedo change.
- Methane feedback: Methane releases in the Arctic further add to the acceleration of warming in the Arctic, further contributing to weaken Arctic methane stores, in a vicious cycle that threatens to escalate into runaway global warming.
- Runaway warming. Accelerating warming further weakens the capability of the seabed to hold the methane that is contained in the form of hydrates and free gas in sediments under the sea, in a vicious cycle that threatens to lead to runaway warming.
What would the impact be of abrupt release of 1Gt of methane in the Arctic, compared to the total global carbon dioxide emissions from fossil-fuel burning, cement manufacture, and gas flaring? Image 22. below gives a rather conservative impact, showing a rapid decline toward a small residual impact as carbon dioxide.
Above graph does not yet include the indirect effect of triggering further releases. This is especially a threat in the Arctic, given the large presence of methane in sediments, the accelerated warming already occurring in the Arctic, the little oxidation that takes place in the Arctic atmosphere, and the time it will take for abruptly released methane to spread away from the Arctic.
The additional warming that this will cause in the Arctic will make that the decline of sea ice and snow cover will take place even more dramatically than is already the case now. There will be a huge warming impact, due to the albedo change caused by decline of snow and ice combined with the warming impact of methane. This threatens to trigger further releases of methane in the Arctic, with their joint impact accumulating as illustrated in the image below.
For more details on above two graphs, also see the page How much time is there left to act?
Dramatic warming will first strike in the Arctic, but will soon spread, threatening to cause heatwaves and firestorms across North America and Siberia, adding additional soot and carbon dioxide in the atmosphere globally, as forests, peat bogs and tundras at higher latitudes burn, threatening to escalate in runaway global warming.
8. Temperature rise
![]() |
Image 24. Global warming, accelerated warming in the Arctic, and runaway global warming |
9. Destruction and Extinction
In the above projection, runaway global warming will catch up with Arctic warming by 2039, resulting in a global temperature increase of 10 degrees Celsius and rising. The heatwaves that will come with such a temperature rise will in itself be enough to cause crop losses at massive scale. Additionally, heatwaves at high latitudes will cause wildfires, e.g. in Siberia, which has a very high soil carbon content (see image below).
![]() |
Image 25. Organic soil carbon content |
![]() |
Image 26. Warming and feedbacks leading to destruction and extinction at massive scale |
10. Ways to avoid catastrophe
It takes a comprehensive climate plan to combine the policies that jointly work most effectively to avoid the catastrophic climate change that has been pictured in the previous chapters.
Such a comprehensive climate plan needs to combine emission reduction policies with policies that can remove pollutants such as carbon dioxide and methane from the atmosphere and oceans. Furthermore, solar radiation management methods to to be prepared to rapidly cool the Arctic.
A comprehensive climate plan will operate along several lines of action simultaneously.
![]() |
Image 27. Energy feebates |
- energy feebates (pictured above) in sectors such as electricity, heating and transport
- feebates in sectors such as agriculture, land use, waste management and construction (pictured below).
![]() |
Image 28. Feebates in sectors such as agriculture, land use and construction |
The revenues are then used to fund rebates on clean construction and on soil supplements containing biochar and olive sand, which will remove carbon dioxide from the atmosphere and store it in buildings, soil, river banks, roads and pavement.
Working separately, yet complimentary, energy feebates and feebates in agriculture and other sectors can dramatically bring down carbon dioxide levels in the atmosphere and oceans; as a result, atmospheric carbon dioxide could be brought back to pre-industral levels of around 280ppm by the end of the century.
![]() |
Image 29. Getting carbon dioxide in the atmosphere back to 280ppm. For further discussion, also see Towards a Sustainable Economy |
Even with these measures, temperatures will keep rising for some time, as excess ocean heat will get transferred to the atmosphere over the years and as aerosols (particularly sulfur) fall away that are currently emitted when fuel is burned and that mask the full wrath of global warming.
Continued warming comes with numerous feedbacks. Combined, these feedbacks threaten to trigger runaway global warming, i.e. warming that will cause mass death, destruction and extinction.
The image below pictures several methods of Arctic methane management that should get high priority, given the threat of hydrate destabilization in the Arctic.
![]() |
Image 31. Arctic Methane Management |
In this case, it makes sense to add fees, e.g., on commercial international flights (in addition to fees that may already have been imposed locally, such as on jetfuel), and to use the reveneus to fund action such as the vessels proposed by Stephen Salter and John Latham, in order to provide much-needed cooling in the Arctic locally during the northern summer.
Fees imposed on commercial international flights could fund solar radiation management, while the two types of feebates described further above will also be most effective in further lines of action, i.e. in Arctic methane management and further action.
Image 32. below pictures feebates that in many cases can be implemented locally on a budget-neutral basis.
![]() |
Image 32. Feebates (yellow lines) |
Text References
1. Potential methane reservoirs beneath Antarctica
Press release University of Bristol (2012)
2. Potential methane reservoirs beneath Antarctica
J. L.Wadham, S. Arndt, S. Tulaczyk, M. Stibal, M. Tranter, J. Telling, G. P. Lis, E. Lawson, A. Ridgwell, A. Dubnick, M. J. Sharp, A. M. Anesio & C. Butler (2012)
3. Methane release from the East Siberian Arctic Shelf and the Potential for Abrupt Climate ChangeJ. L.Wadham, S. Arndt, S. Tulaczyk, M. Stibal, M. Tranter, J. Telling, G. P. Lis, E. Lawson, A. Ridgwell, A. Dubnick, M. J. Sharp, A. M. Anesio & C. Butler (2012)
Natalia Shakhova and Igor Semiletov (2010)
Presentation at Symposium, November 30, 2010
http://symposium2010.serdp-estcp.org/content/download/8914/107496/version/3/file/1A_Shakhova_Final.pdf
4. On carbon transport and fate in the East Siberian Arctic land–shelf–atmosphere system
Semiletov et al. (2012)
http://iopscience.iop.org/1748-9326/7/1/015201
5. Anomalies of methane in the atmosphere over the East Siberian shelf: Is there any sign of methane leakage from shallow shelf hydrates?
Shakhova, Semiletov, Salyuk and Kosmach (2008)
EGU General Assembly 2008
EGU General Assembly 2008
http://www.cosis.net/abstracts/EGU2008/01526/EGU2008-A-01526.pdf
6. Working Group I: The Physical Science Basis, Table 2.14
IPCC, Climate Change 2007
http://www.ipcc.ch/publications_and_data/ar4/wg1/en/ch2s2-10-2.html
7. Improved Attribution of Climate Forcing to Emissions
Drew Shindell et al. (2009)
http://www.sciencemag.org/content/326/5953/716.abstract
8. Poster at AGU 2011
Arctic Methane Emergency Group (AMEG), artwork by Sam Carana (2011)
http://arctic-news.blogspot.com/p/agu-poster.html
9. Working Group I: The Physical Science Basis, FAQ 10.3
IPCC, Climate Change 2007
http://www.ipcc.ch/publications_and_data/ar4/wg1/en/faq-10-3.html
10. Working Group I: The Scientific Basis, 4.1.1 Sources of Greenhouse Gases
IPCC, TAR (2001)
http://www.ipcc.ch/ipccreports/tar/wg1/130.htm#tab41a
11. Atmospheric Chemistry and Greenhouse Gases, Executive Summary
IPCC, TAR, 04 (2001)
http://www.ipcc.ch/ipccreports/tar/wg1/128.htm
12. Interactions with Aerosols Boost Warming Potential of Some Gases
NASA (2009)
http://www.giss.nasa.gov/research/news/20091029/
13. Reactive greenhouse gas scenarios: Systematic exploration of uncertainties and the role of atmospheric chemistry
Prather et al. (2012)
http://www.agu.org/pubs/crossref/2012/2012GL051440.shtml
14. Hydroxyl radical buffered by isoprene oxidation over tropical forests
Taraborelli et al (2012)
http://www.nature.com/ngeo/journal/vaop/ncurrent/full/ngeo1405.html
15. Have sudden large releases of methane from geological reservoirs occurred since the Last Glacial Maximum, and could such releases occur again?
Euan G. Nisbet (2002)
http://rsta.royalsocietypublishing.org/content/360/1793/581.abstract
16. How much time is there left to act?
Sam Carana (2012)
http://arctic-news.blogspot.com/p/how-much-time-is-there-left-to-act.html
17. First drilling subsea permafrost in the southeastern Laptev Sea, the East Siberian Arctic Shelf: results and challenges
Semiletov et al., Geophysical Research Abstracts (2012)
http://meetingorganizer.copernicus.org/EGU2012/EGU2012-3913.pdf
18. Submarine pingoes: Indicators of shallow gas hydrates in a pockmark at Nyegga, Norwegian Sea.
Hovland et al., Marine Geology 228 (2006) 15–23
http://www.sciencedirect.com/science/article/pii/S0025322705003968
Images references
1. Methane levels, March 19, 2013.
adapted by Sam Carana from NOAA, 2013
http://www.noaa.gov/
2. Global distribution of atmospheric methane
adapted by Sam Carana from NOAA, May 2006
http://www.noaa.gov/
3. Methane at up to 2241 ppb at 742 mb on January 23, 2013
from: Dramatic increase in methane in the Arctic in January 2013, by Sam Carana
http://arctic-news.blogspot.com/2013/02/dramatic-increase-in-methane-in-the-arctic-in-january-2013.html
4. IASI methane levels March 1-10, 2013, by Leonid Yurganov, against NSIDC sea ice concentration map March 12, 2013
from: Record methane in the Arctic early March 2013, by Sam Carana
http://arctic-news.blogspot.com/2013/03/record-methane-in-arctic-early-march-2013.html
5. Antarctic glacier, by Jemma Wadham
Potential methane reservoirs beneath Antarctica
Press release University of Bristol (2012)
12. Big changes in Arctic within years
http://arctic-news.blogspot.com/2012/10/big-changes-in-arctic-within-years.html
13. M. G. Flanner et al. (2011), Radiative forcing and albedo feedback from the Northern Hemisphere cryosphere between 1979 and 2008.
http://www.nature.com/ngeo/journal/v4/n3/full/ngeo1062.html
14. M. G. Flanner et al. (2011), Radiative forcing and albedo feedback from the Northern Hemisphere cryosphere between 1979 and 2008.
http://www.nature.com/ngeo/journal/v4/n3/full/ngeo1062.html
15. Insolation on the Northern Hemisphere
from: Pidwirny, M. (2006). "Earth-Sun Relationships and Insolation". Fundamentals of Physical Geography, 2nd Edition.
physicalgeography.net/fundamentals/6i.html
16. Global hydroxyl levels day and night
From: Taraborelli et al (2012), Hydroxyl radical buffered by isoprene oxidation over tropical forests
http://www.nature.com/ngeo/journal/vaop/ncurrent/full/ngeo1405.html
17. Shallow waters in the Arctic Ocean
Image adapted by Sam Carana from NOAA image
http://ngdc.noaa.gov
18. Shallow waters of the East Siberian Arctic Shelf (ESAS)
On carbon transport and fate in the East Siberian Arctic land–shelf–atmosphere system
Semiletov et al. (2012)
http://iopscience.iop.org/1748-9326/7/1/015201
19. Warming of coastal waters - Mean SST on August 22. 2007, in degrees Celsius
Produced by Sam Carana from:
http://esrl.noaa.gov/
20. Submarine pingoes: Indicators of shallow gas hydrates in a pockmark at Nyegga, Norwegian Sea.
Hovland et al., Marine Geology 228 (2006) 15–23
http://www.sciencedirect.com/science/article/pii/S0025322705003968
21. Three kinds of warming and feedbacks
Sam Carana (2012)
http://arctic-news.blogspot.com/2012/08/diagram-of-doom.html
22. Abrupt release of 1Gt of methane
Sam Carana (2012), How much time is there left to act
http://arctic-news.blogspot.com/p/how-much-time-is-there-left-to-act.html
23. The accumulating impact of methane releases in the Arctic
Sam Carana (2012), How much time is there left to act
http://arctic-news.blogspot.com/p/how-much-time-is-there-left-to-act.html
24. Global warming, accelerated warming in the Arctic, and runaway global warming
Sam Carana (2013) - see also: How much will temperatures rise?
http://arctic-news.blogspot.com/2013/04/how-much-will-temperatures-rise.html
25. Organic soil carbon content
from: EUR 21855 EN - soil organic carbon European Commission (2011) http://ies.jrc.ec.europa.eu/uploads/fileadmin/Documentation/JRC-CC-COP17.pdf.pdf
26. Warming and feedbacks leading to destruction and extinction at massive scale
Sam Carana (2013) - see also: An Effective and Comprehensive Climate Plan
http://climateplan.blogspot.com/2013/01/an-effective-and-comprehensive-climate-plan.html
27. Energy feebates
Towards a sustainable economy, by Sam Carana (2011)
http://feebates.blogspot.com/2011/09/towards-sustainable-economy.html
28. Feebates in sectors such as agriculture, land use and construction
Towards a sustainable economy, by Sam Carana (2011)
http://feebates.blogspot.com/2011/09/towards-sustainable-economy.html
29. Getting carbon dioxide in the atmosphere back to 280ppm
Towards a sustainable economy, by Sam Carana (2011)
http://feebates.blogspot.com/2011/09/towards-sustainable-economy.html
30. Diagram of Doom and 3-part action plan
Sam Carana (2013)
http://climateplan.blogspot.com
31. Arctic Methane Management
Sam Carana (2012)
http://arctic-news.blogspot.com/p/arctic-methane-management.html
32. Feebates
Towards a sustainable economy, by Sam Carana (2011)
http://feebates.blogspot.com/2011/09/towards-sustainable-economy.html
6. Working Group I: The Physical Science Basis, Table 2.14
IPCC, Climate Change 2007
http://www.ipcc.ch/publications_and_data/ar4/wg1/en/ch2s2-10-2.html
7. Improved Attribution of Climate Forcing to Emissions
Drew Shindell et al. (2009)
http://www.sciencemag.org/content/326/5953/716.abstract
8. Poster at AGU 2011
Arctic Methane Emergency Group (AMEG), artwork by Sam Carana (2011)
http://arctic-news.blogspot.com/p/agu-poster.html
9. Working Group I: The Physical Science Basis, FAQ 10.3
IPCC, Climate Change 2007
http://www.ipcc.ch/publications_and_data/ar4/wg1/en/faq-10-3.html
10. Working Group I: The Scientific Basis, 4.1.1 Sources of Greenhouse Gases
IPCC, TAR (2001)
http://www.ipcc.ch/ipccreports/tar/wg1/130.htm#tab41a
11. Atmospheric Chemistry and Greenhouse Gases, Executive Summary
IPCC, TAR, 04 (2001)
http://www.ipcc.ch/ipccreports/tar/wg1/128.htm
12. Interactions with Aerosols Boost Warming Potential of Some Gases
NASA (2009)
http://www.giss.nasa.gov/research/news/20091029/
13. Reactive greenhouse gas scenarios: Systematic exploration of uncertainties and the role of atmospheric chemistry
Prather et al. (2012)
http://www.agu.org/pubs/crossref/2012/2012GL051440.shtml
14. Hydroxyl radical buffered by isoprene oxidation over tropical forests
Taraborelli et al (2012)
http://www.nature.com/ngeo/journal/vaop/ncurrent/full/ngeo1405.html
15. Have sudden large releases of methane from geological reservoirs occurred since the Last Glacial Maximum, and could such releases occur again?
Euan G. Nisbet (2002)
http://rsta.royalsocietypublishing.org/content/360/1793/581.abstract
16. How much time is there left to act?
Sam Carana (2012)
http://arctic-news.blogspot.com/p/how-much-time-is-there-left-to-act.html
17. First drilling subsea permafrost in the southeastern Laptev Sea, the East Siberian Arctic Shelf: results and challenges
Semiletov et al., Geophysical Research Abstracts (2012)
http://meetingorganizer.copernicus.org/EGU2012/EGU2012-3913.pdf
18. Submarine pingoes: Indicators of shallow gas hydrates in a pockmark at Nyegga, Norwegian Sea.
Hovland et al., Marine Geology 228 (2006) 15–23
http://www.sciencedirect.com/science/article/pii/S0025322705003968
Images references
1. Methane levels, March 19, 2013.
adapted by Sam Carana from NOAA, 2013
http://www.noaa.gov/
2. Global distribution of atmospheric methane
adapted by Sam Carana from NOAA, May 2006
http://www.noaa.gov/
3. Methane at up to 2241 ppb at 742 mb on January 23, 2013
from: Dramatic increase in methane in the Arctic in January 2013, by Sam Carana
http://arctic-news.blogspot.com/2013/02/dramatic-increase-in-methane-in-the-arctic-in-january-2013.html
4. IASI methane levels March 1-10, 2013, by Leonid Yurganov, against NSIDC sea ice concentration map March 12, 2013
from: Record methane in the Arctic early March 2013, by Sam Carana
http://arctic-news.blogspot.com/2013/03/record-methane-in-arctic-early-march-2013.html
5. Antarctic glacier, by Jemma Wadham
Potential methane reservoirs beneath Antarctica
Press release University of Bristol (2012)
Sam Carana (2012)
http://geo-engineering.blogspot.com/2012/6/earth-at-boiling-point.html
7. Arctic Ocean with predicted deposits of CH4 hydrates shown in blue
On carbon transport and fate in the East Siberian Arctic land–shelf–atmosphere system
Semiletov et al. (2012)
http://iopscience.iop.org/1748-9326/7/1/015201
8. IPCC has upgraded methane's GWP (global warming potential) several times over the years
from: Video and poster - methane in the Arctic, by Sam Carana (2012)
http://arctic-news.blogspot.com/2012/05/video-and-poster-methane-in-arctic.html
9. Methane's Global Warming Potential (GWP)
from: Video and poster - methane in the Arctic, by Sam Carana (2012)
http://arctic-news.blogspot.com/2012/05/video-and-poster-methane-in-arctic.html
10. Methane's Local Warming Potential (LWP)
Sam Carana (2013)
11. Estimates of Total Antarctic Land Ice Changes
from: Is Antarctica losing or gaining ice?
http://www.skepticalscience.com/antarctica-gaining-ice.htm
http://geo-engineering.blogspot.com/2012/6/earth-at-boiling-point.html
7. Arctic Ocean with predicted deposits of CH4 hydrates shown in blue
On carbon transport and fate in the East Siberian Arctic land–shelf–atmosphere system
Semiletov et al. (2012)
http://iopscience.iop.org/1748-9326/7/1/015201
8. IPCC has upgraded methane's GWP (global warming potential) several times over the years
from: Video and poster - methane in the Arctic, by Sam Carana (2012)
http://arctic-news.blogspot.com/2012/05/video-and-poster-methane-in-arctic.html
9. Methane's Global Warming Potential (GWP)
from: Video and poster - methane in the Arctic, by Sam Carana (2012)
http://arctic-news.blogspot.com/2012/05/video-and-poster-methane-in-arctic.html
10. Methane's Local Warming Potential (LWP)
Sam Carana (2013)
11. Estimates of Total Antarctic Land Ice Changes
from: Is Antarctica losing or gaining ice?
http://www.skepticalscience.com/antarctica-gaining-ice.htm
12. Big changes in Arctic within years
http://arctic-news.blogspot.com/2012/10/big-changes-in-arctic-within-years.html
13. M. G. Flanner et al. (2011), Radiative forcing and albedo feedback from the Northern Hemisphere cryosphere between 1979 and 2008.
http://www.nature.com/ngeo/journal/v4/n3/full/ngeo1062.html
14. M. G. Flanner et al. (2011), Radiative forcing and albedo feedback from the Northern Hemisphere cryosphere between 1979 and 2008.
http://www.nature.com/ngeo/journal/v4/n3/full/ngeo1062.html
15. Insolation on the Northern Hemisphere
from: Pidwirny, M. (2006). "Earth-Sun Relationships and Insolation". Fundamentals of Physical Geography, 2nd Edition.
physicalgeography.net/fundamentals/6i.html
16. Global hydroxyl levels day and night
From: Taraborelli et al (2012), Hydroxyl radical buffered by isoprene oxidation over tropical forests
http://www.nature.com/ngeo/journal/vaop/ncurrent/full/ngeo1405.html
17. Shallow waters in the Arctic Ocean
Image adapted by Sam Carana from NOAA image
http://ngdc.noaa.gov
18. Shallow waters of the East Siberian Arctic Shelf (ESAS)
On carbon transport and fate in the East Siberian Arctic land–shelf–atmosphere system
Semiletov et al. (2012)
http://iopscience.iop.org/1748-9326/7/1/015201
19. Warming of coastal waters - Mean SST on August 22. 2007, in degrees Celsius
Produced by Sam Carana from:
http://esrl.noaa.gov/
20. Submarine pingoes: Indicators of shallow gas hydrates in a pockmark at Nyegga, Norwegian Sea.
Hovland et al., Marine Geology 228 (2006) 15–23
http://www.sciencedirect.com/science/article/pii/S0025322705003968
21. Three kinds of warming and feedbacks
Sam Carana (2012)
http://arctic-news.blogspot.com/2012/08/diagram-of-doom.html
22. Abrupt release of 1Gt of methane
Sam Carana (2012), How much time is there left to act
http://arctic-news.blogspot.com/p/how-much-time-is-there-left-to-act.html
23. The accumulating impact of methane releases in the Arctic
Sam Carana (2012), How much time is there left to act
http://arctic-news.blogspot.com/p/how-much-time-is-there-left-to-act.html
24. Global warming, accelerated warming in the Arctic, and runaway global warming
Sam Carana (2013) - see also: How much will temperatures rise?
http://arctic-news.blogspot.com/2013/04/how-much-will-temperatures-rise.html
25. Organic soil carbon content
from: EUR 21855 EN - soil organic carbon European Commission (2011) http://ies.jrc.ec.europa.eu/uploads/fileadmin/Documentation/JRC-CC-COP17.pdf.pdf
26. Warming and feedbacks leading to destruction and extinction at massive scale
Sam Carana (2013) - see also: An Effective and Comprehensive Climate Plan
http://climateplan.blogspot.com/2013/01/an-effective-and-comprehensive-climate-plan.html
27. Energy feebates
Towards a sustainable economy, by Sam Carana (2011)
http://feebates.blogspot.com/2011/09/towards-sustainable-economy.html
28. Feebates in sectors such as agriculture, land use and construction
Towards a sustainable economy, by Sam Carana (2011)
http://feebates.blogspot.com/2011/09/towards-sustainable-economy.html
29. Getting carbon dioxide in the atmosphere back to 280ppm
Towards a sustainable economy, by Sam Carana (2011)
http://feebates.blogspot.com/2011/09/towards-sustainable-economy.html
30. Diagram of Doom and 3-part action plan
Sam Carana (2013)
http://climateplan.blogspot.com
31. Arctic Methane Management
Sam Carana (2012)
http://arctic-news.blogspot.com/p/arctic-methane-management.html
32. Feebates
Towards a sustainable economy, by Sam Carana (2011)
http://feebates.blogspot.com/2011/09/towards-sustainable-economy.html